Research Overview
Multimodal Nonlinear Imaging and nonlinear phototherapy
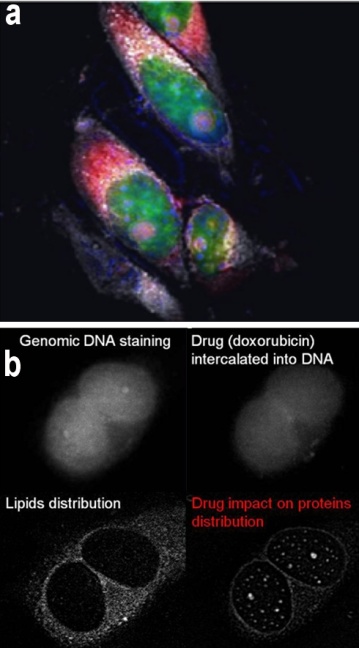
(a) Merged imaging of HeLa cells: Proteins (blue), RNA (red), DNA (green) and Lipids (grey). (b) HeLa imaging after 24 hours of doxorubicin treatment.
Multimodal Nonlinear imaging based on Coherent Anti-Stokes Raman Scattering (CARS), Second Harmonic / Sum Frequency Generation (SHG/SFG) and Two Photon Excited Fluorescence (TPEF), is an important technique among that used in biomedical studies. In this optical combination CARS modality enables selective visualization of chemical bonds and is used for selective label-free imaging of native cellular proteins and lipids. The SHG/SFG are the excellent modalities for visualization of collagen fibrils in the tissue, extracellular matrix and cellular membranes. Finally, TPEF bridges these types of label-free imaging of cellular macromolecules together with fluorescence detection of specific probes in the samples.Multimodal Nonlinear imaging can be considered as one of the most powerful non-invasive tools for biomolecular mapping in the live cells and tissues. We use it studying changes in biomolecular composition of live cells during cell cycle, apoptosis process and in the course of drug treatment.
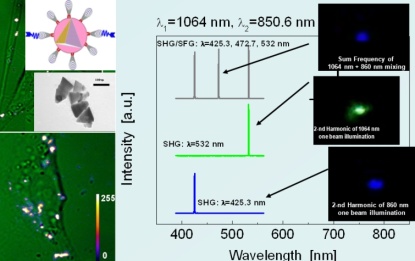
SHG and SFG imaging of ZnO nanoparticles in live HeLa cells
We are also developing new methods of monitoring the intracellular distribution of nanoparticles with high non-linearity based on SHG and SFG for further application of converted light in therapeutic applications.Natural intracellular molecules such as proteins, nucleic acids, lipids and even water, which demonstrate optical non-linear properties, can also be successfully employed for optical upconversion and then trigger photoactivation of different processes. We utilized thisphenomenon for novel nonlinear Photodynamic therapy (PDT) concept. A major limitation of traditional PDT is insufficient penetration of visible (used for PDT drug excitation)light through the tissue, which makes treatment of large or distant tumors not effective. At the same time near-infrared (NIR) light can easily penetrate into the tissue and being up-converted in-situ by natural cellular biomolecules in visible and UV through the CARS/FWM and SHG/SFG processes, can be successively used for photoactivation of most established PDT drugs.
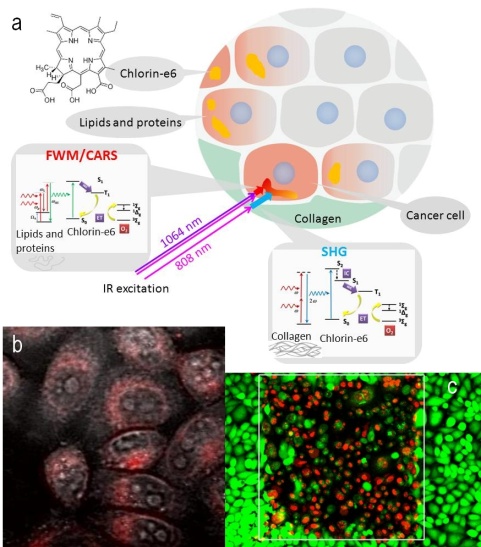
Scheme of in situ nonlinear photon conversion in cancer tissue (a), co-localization of cytoplasmic proteins and Ce6 in CARS/TPEF imaging (b), and result of PDT through CARS/TPEF photon conversion
Application of CARS imaging for condensed matter physics studies
CARS imaging of liquid crystals (LC) is another exciting area of application of non-linear imaging technique. Polarized CARS signal of LC is sensitive to orientation of anisotropic molecules in liquid crystals, or director. This property allows us to produce spatial mapping of the director structures, and, therefore to visualize different types of defects in LC. Because of high non-linearity of LC leading to (i) excellent imaging contrast and (ii) short times of signal integration and imaging acquisition (less than 1 s), this technique might allow to study temporal dynamics of the director structures.
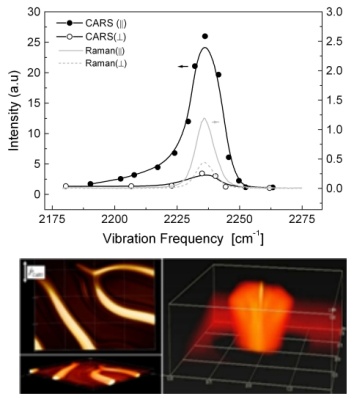
Raman and CARS spectra of of the CN bond of 8CB and 3D CARS imaging of cholesteric finger structure in a chiral nematic mixture (left) and confocal domains in smectic layers (right) of liquid crystal at 2235 cm
Raman Micro-spectrometry
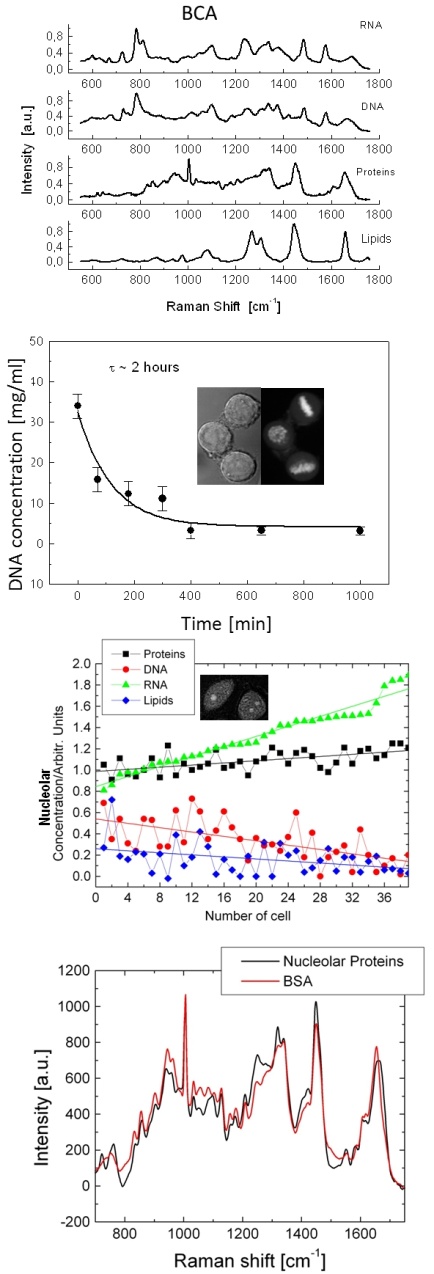
Raman spectra of biomolecular components used in BCA. Relaxation of DNA concentration after mitosis. BCA result obtained for data set containing Raman spectra of 34 nucleoli of different cells. Overlapped Raman spectrum of Bovine Serum Albumin and protein component obtained by BCA of nucleolus in live HeLa cell.
While non-linear imaging is excellent for mapping of molecular distributions in 2D and 3D objects, Raman micro-spectrometry is efficient tool for site specific quantitive molecular analysis. In combination with the Biomolecular Analysis (BCA), this technique is the most powerful tool for single cell assay, and can interrogate the molecular content of distinct cellular domains in-situ. Using this tool we are investigating the processes in single live cells such as relaxation of chromatin after mitosis, monitoring the synthesis of rRNA and p in nucleolus and local changes of subcellular molecular environment during both physiologic and pathologic processes. We also study an effect of chemical fixation on biomolecular composition of individual domains of the cell nucleus We are also developing methods for targeting the specific intracellular domains by Raman markers, including Resonant Raman markers, SERS markers and exogenous markers with characteristic spectral bands (e.g. C-D, C=N, C=C) in bio-silent spectral Raman region.
Anti-Stokes Fluorescence Confocal Microscopy (Temperature Mapping)
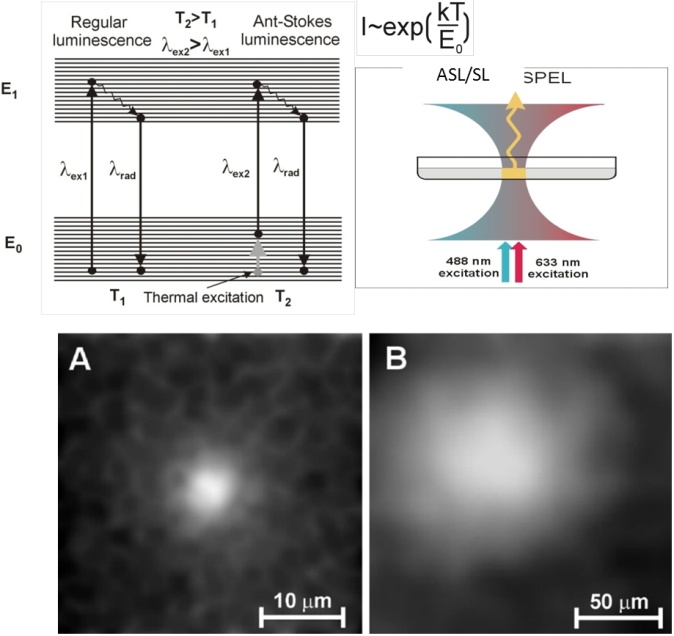
Anti-Stokes images of heated area of polystyrene films with dispersed Rh640 as a probe dye and YbLiF3 nanoheaters as a heating agent: (A) is an image of sample with layered structure; (B) is a picture obtained for film containing uniformly distributed NPs.
Anti-Stokes luminescence is caused by the optical excitation when absorption of photons with lower energy from thermally populated vibrational-rotational states of the ground electronic state. Since the population of the upper vibrational levels of the ground state is temperature dependent (based on Boltzmann distribution), the ASL intensity is extremely temperature dependent. The anti-Stokes fluorescence technique is particularly suitable for micro- and sub-micro characterization of in-plane thermal transport in thin films and allows for both transient as well as steady-state regimes of characterization. The sub-microscale length regime, falling between sub-continuum and continuum transport physics, is of particular importance, as recent work has shown that material processing to create domain sizes in this regime offers substantial, though highly complex, improvements in thermoelectric performance.
Optical Trapping
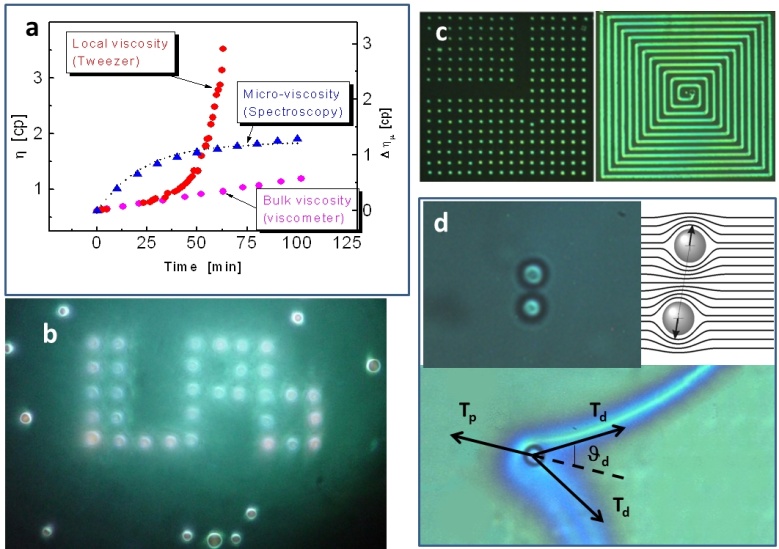
Application of Laser Tweezers for sol-gel micro-viscosity monitoring (a), for fast scanning single laser beam multi-trap beads manipulation (b) dynamically reconfigurable photonic structures (c) and colloidal interaction forces measurements in LC (d).
Since optical tweezers was introduced by Ashkin in 1970, the ability of this tool to apply pico-Newton-level forces to micron-sized particles while simultaneously measuring displacement with nanometer-level precision is now routinely applied to the study of molecular motors at the single-molecule level, the physics of colloids and mesoscopic systems, and the mechanical properties of polymers and biopolymers. We used this technique for variety studies in the physics of liquid crystals, sol-gel processes and biological objects.
Lasers
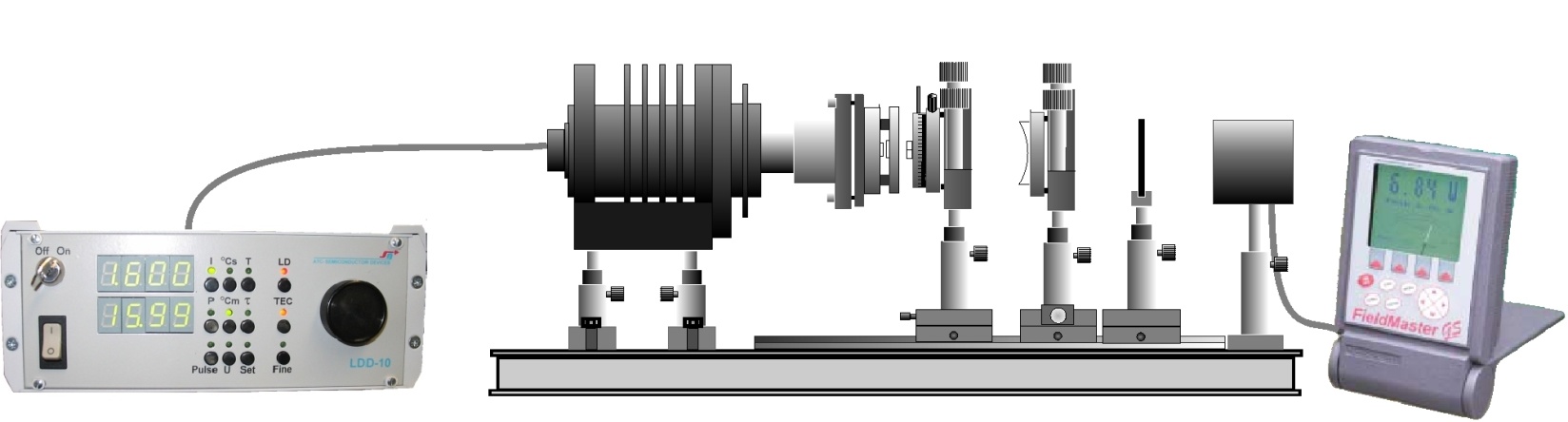
We have developed versatile laser/course materials designed to teach University students to the latest in solid-state laser technology and a wide range of physical principles.
Laboratory laser experiments included in the Laser Kit Manual.
- Experiment #1: Measurement of the time relaxation of the upper laser level of Nd3+:KGd(WO4)2
- Experiment #2: Obtaining CW laser operation
- Experiment #3: Single mode laser operation and resonator stability
- Experiment #4: Measurement of laser threshold and output power at CW laser operation
- Experiment #5: Passive Q-switched regime of laser operation
- Experiment #6: Passive Q-switched regime of laser operation
- Experiment #7: Intracavity CW second harmonic generation
- Experiment #8: Intracavity second harmonic generation using Q-switched laser operation
- Experiment #9: Intracavity Raman self-conversion using Q-switched laser operation
Nonlinear Optical Effects in Organic Polymers
Our research program in this area represents study of new nonlinear optical phenomena, design and development of nonlinear optical polymers, study of their ultrastructure, and dynamics of nonlinear optical interactions. Our current focus is on the study of photorefractivity which is a second order effect and various third-order nonlinear optical manifestations in organic: inorganic hybrids as well as in artificially nanostructured (such as photonic crystal) media. In the area of photorefractivity, we are using a nanophotonic approach to produce novel hybrid photorefractive media, with a specific emphasis on covering telecommunication wavelengths of 1.3 and 1.55 microns. One example is the use of a polymeric nanocomposite in which quantum dots are used as photosensitizers to generate charge carriers; the polymer medium is used for charge separation to build a space–charge field, and liquid crystal nanodroplets are used to generate a refractive index charge: electro-optic effect produced: the space-charge field. We study the fundamental processes of charge-generation, charge separation, mobility of carriers (time of flight) and the space-charge field. The photorefractive effect and the dynamics of buildup and decay are investigated: asymmetric two-beams coupling and four wave mixing. We have demonstrated, for the first time, photorefractive operations in a polymeric media at 1.3 and 1.55 um
Third-order nonlinear optical effects are being probed: femtosecond time-resolved pump-probe experiments, Z-scan, Kerr-gate, degenerate four waves mixing and third harmonic generation. We recently reported the phenomena of nonlinear Rayleigh-Bragg scattering. Also reported recently were phase-matched third-harmonic in a photonic crystal medium
Multiphoton Processes and Applications
The research program in this area focuses on the development of multifunctional structures with enhanced two-photon, three-photon and four-photon absorption cross-sections as well as efficient up-converted emissions. Theoretical modeling and synthesis are used together to produce such materials. The dynamics of these multiphoton processes are investigated using femtosecond time-resolved four wave mixing, transient absorption, Kerr-gate and Z-scan. We have developed a novel method using femtosecond white-light continuum for obtaining two-photon spectra. The applications of two-photon and three-photon processes to multiphoton multicolor confocal microscopy, optical data storage and two-photon photodynamic therapy is very exciting directions which are being developed in our laboratory. Other applications being pursued are two-photon, three photon and four-photon pumped up-conversion lasing, optical power limiting, optical phase conjugation and optical power stabilization. We recently reported even three-photon and four-photon pumped population inversion and subsequent stimulated emissions. Another exciting prospect being developed is two-photon lithography and two-photon holography for three-dimensional optical circuitry and for photonic crystal fabrication
Another are of recent activities is the studies of multiphoton absorption in quantum dots. We have demonstrated efficient two and three photon absorption processes in quantum dots covering a broad spectra range from visible to IR.
Metaphotonics and Chiral Photonics
In a very broad sense, metaphotonics deals with manipulation of electro-magnetic fields using electric dipole and quadrupole, and magnetic dipole interactions as well as their cross-coupling in nanoengineered (meta) materials. In traditional approaches to the optics of natural media and structures employed in photonics, electric interactions and electric field control play the dominant role, leading to dipole controlled propagation and light–matter interactions, positive refractive index, electrooptic effects, etc.]. On the other hand, the combination of electric and magnetic interactions, inherent to metaphotonics, allows for manipulating the value of the refractive index, including near-zero and negative values, as well as its gradient, leading to phenomena such as sub-wavelength resolution, cloaking, slow light, magnetic field switching and optical control of magnetism. All of this is made possible by physics-guided nanoengineering of appropriate artificial media. Apart from astounding new physical insights, the metaphotonics approach can also produce new breakthrough technologies. Potential applications of metaphotonics range from optical communications to solar energy harvesting, to sensor technology, to biophotonics. Nonlinear metaphotonics,i.e.,self-action and frequency mixing of light in nanoengineered materials, possessing second- and third-order optical nonlinearities, while still in its infancy, can provide even further novel means of manipulating light. The underlying physical mechanism of many (but not all) of the above mentioned phenomena is the enhancement of the role of magnetic coupling using either artificial nanoresonators or chiral molecular and nanocomposite media.
In our program, we are using a chiral approach involving a nanocomposite of a multiscale modeling based chiral polymer nanocomposite containing plasmonic, excitonic and magnetic nanoinclusion. Negative index media (NIM) produce a large number of novel optical manifestations and thus form a hot topic. All approaches in the past have involved either manipulation of photonic band structure or producing negative dielectric permittivity and magnetic permeability. We are taking a novel chemical approach of using coupled electric and magnetic resonances in a chiral medium to achieve negative refraction. Our approach uses molecular chirality, amplification of chirality using supramolecular organizations and inclusions of plasmonic and excitonic nanostructures.
Another major focus is study of third-order nonlinear optical effects in chiral media. We are studying both two-photon absorption and intensity dependent refractive index. Our experimental approach is Z-scan. We have developed new methods of photoelastic modulation coupled with Z-scan, to study chirality effects and are building theoretical understand of these processes. With magnetic nanoinclusions in a chiral medium, we have shown enhancement of magnetic interactions to produce modulation and control of magneto-optic properties
Biophotonics
This is a new frontier of science which utilizes light for medical diagnostics, optical biosensing, laser tissue engineering, and light activated therapy. Biophotonics forms a major research thrust of our program. On the fundamental level, our program involves the study of light activated processes at both cellular and tissue levels. Optical imaging using near field microscopy, confocal microscopy and optical coherence tomography is used to study structure and dynamics both at cellular and tissue levels. An important aspect of our program is the use of nonlinear optical processes such as second harmonic generation, sum frequency generation, two-photon excitation and coherent anti-stokes Raman scattering (CARS). CARS provide a powerful method for molecular imaging: using a Raman vibration as a specific biomarker. CARS imaging, together with imaging using the nonlinear process of sum frequency generation, are also being used for both in-vitro and in-vivo studies. We are developing novel two-photon fluorophores; water dispersible and surface functionalized quantum dots; and up-converting rare-earth containing nanoparticles, as well as CARS probes for multispectral bioimaging
Multimodal optical imaging is being utilized to create a fundamental insight at molecular level on such biological processes as gene expression, cell cycle progression, malignant transformations and cell death. For instance, CARS and two-photon excited fluorescence (TPEF) imaging has been recently introduced: our group for monitoring of subcellular distribution of major types of macromolecules (proteins, lipids, DNA and RNA) throughout the cell cycle and during the propagation of apoptosis. Furthermore, a combination of these imaging techniques with quantitative Raman spectroscopy approach enables for both imaging of macromolecules and nearly simultaneous measurements of their local concentrations in the subcellular sites of interest, such as cytoplasmic organelles and compartments in the cell nucleus. The combination of Raman spectroscopy and CARS/TPEF imaging is aimed at identification of the macromolecular distribution signatures characterizing various physiological and pathological processes in the cell and for a long term monitoring of intracellular distribution of macromolecules. Nowadays, it becomes increasingly clear that the cells affected: metabolic disorders, pathogenesis, infectious diseases, malignant transformations, etc., exhibit a deregulation of synthesis and intracellular distribution of major groups of macromolecules and CARS/TPEF imaging together with Raman microspectrometry can provide information essential for molecular diagnostics and drugs development.
Fluorescence based life time imaging (FLIM), Forster resonance energy transfer (FRET), fluorescence recovering after photobleaching (FRAP) are being used to understand protein association, interaction and conformational dynamics. In particular, the FLIM approach probes the molecular environment of the excited fluorophore, at the nanoscale, far beyond constrains of optical resolution: our group FLIM has recently been introduced for sensing of the transformation of the nuclear environment throughout the cell cycle
The in-vitro and in-vivo bioimaging studies are also targeted to create an understanding of drug biodistribution, cellular interaction and localization, molecular mechanism of drug action and real time monitoring of a therapeutic process or a disease progression.
In the area of biosensing, the focus is intracellular sensing using a variety of molecular probes and nanoprobes. Another area of analyte and bacterial detection being developed is a compact advanced multiparameter flow cytometer providing some unique capabilities for clinical diagnostics. Another area of activity is the use of laser tweezers and scissors for probing cellular interactions and producing biological manipulation. Laser tweezer Raman studies are being used to obtain Raman spectra of a specific cellular location in order to probe the structure and dynamics within a cell.
A new area of biophotonics is in the development of Nanomedicine, where the combination of Biophotonics with Nanotechnology provides unique opportunities for multimodal imaging, diagnostics, sensing, real-time monitoring of a therapy, and targeted drug delivery. Nanomedicine is further discussed below.
A new concept in target-specific optical diagnostic and therapy is a "Nanoclinic" which is a silica bubble 20 40 nm in size containing various probes and drug delivery system. The surface of this silica bubble is functionalized with targeting groups to carry the nanoclinic to a specific site where the probes and the drug delivery system can be externally activated. We are exploring the application of up-converting and two-photon absorbing materials for photodynamic therapy treatment of deep tumors. We are also using organically modified silica nanoparticles as non-viral vectors for gene delivery.
Nanophotonics
Nanophotonics defined as nanoscale optical science and technology is an emerging field. It offers challenging opportunities for studying fundamental processes of interaction between the radiation field and matter on a scale much smaller than the wavelength of radiation as well as for design of novel nanostructured optical materials, synthesis and processing. Furthermore, the use of such confined interaction to spatially localize photochemical processes also offers exciting opportunities for nanofabrication. Nanophotonics is of considerable technological significance.
Our comprehensive program consists of three parts: (i) nanoscale confinement of radiation to control the propagation properties of radiation and its interaction with matter; (ii) nanoscale confinement of matter and domains to control the optical resonances (band gap) and excitation dynamics; and (iii) nanoscale confinement of photoprocesses to control spatial confinement of optically induced changes useful for optical memory applications and nanofabrication. Nonlinear optical interactions involving nanoscale confinement of radiation are theoretically analyzed and experimentally probed using a near-field geometry. Nanoscale confined optical domains to control excitation dynamics and energy transfer and to produce photon localization are illustrated: examples of new nanostructured materials such as rare-earth doped glasses, multiphasic inorganic: organic hybrid nano-composites, quantum dots and photonic band gap materials.
We developed a novel concept of nanostructured multiphasic composite materials in which each phase performs a distinct photonic function, yet the material is optically transparent because the phase separation is on nanometer size scale. These materials are, therefore, a new generation of multifunctional materials that could contribute to the development of smart materials. Hybrid materials developed in our laboratory combine the merits of two distinct classes of materials: organic and inorganic: using novel chemical processing. They also produce new functionality.
We also have major efforts on development of quantum dots, rods and multipods and study of optical transitions and excitation dynamics. We are also producing plasmonic (metallic) nanostructures and studying field enhancement patterns.
New approaches and novel manifestations of phase-matching for harmonic generation in nonlinear photonic crystal are being developed. For studying quantum optics in photonic crystals microcavities, different methods of fabrication of microcavities and extended defects for optical guiding are being developed. Photon localization, slow light and negative index manifestations are also being investigated.
The applications of nanophotonics, which we are working on, utilize spatially localized photochemistry using a near-field excitation for nanofabrication and nanoscale memory. Another is the use of nonlinear optical methods in a near field geometry for non-destructive evaluation of junctions and circuits, as well as of biological species with sub-optical wavelength (~50 nm) resolution.
A major direction for nanophotonics currently being pursued is multiphoton upconversion in rare-earth doped nanocrystals with hierarchical core-shell nanoarchitecture. We have shown that stepwise multiphoton processes in rare-earth ion doped nanocrystals, through sequential absorption among their ladder-like f-orbital states, can produce upconversion of low-intensity cw light sources, and thus can convert deep-tissue penetrating IR light to visible or UV light on site and on demand. We have introduced the term optical nanotransformers for these photon converters. They allow multiphoton microscopy and therapy to be effected with an inexpensive cw light source, as opposed to a high peak power femtosecond light source, often required for two-photon microscopy using organic dyes that undergo two-photon absorption through a virtual state. Recently, we demonstrated, using a novel multistep energy transfer in organic dye-sensitized core-shell architecture, that multiphoton upconversion can be achieved with nanotransformers even with a low power cw lamp source.